TECHNOLOGY INITIATIVES
Drives and
Controls
Axial
Flux Direct Drive Motors
During the early stages of the Initiative, several members visited Magdalena Ridge Observatory, and were given
a very thorough tour and explanation of the modern, 2.4-meter
alt-az telescope by its Chief Engineer, Elwood Downey, and its
Lead Engineer from EOS Technologies, Kevin Harris. The 2.4 meter
MRO
telescope employs direct drives. The telescope itself acts as
the bearings and “frames” for the azimuth and altitude motors. A
ring of permanent magnets in both altitude and azimuth are the
motors’ rotors, and similar rings of coils that act as the
stators. Magnetic forces between the rotor and stator move the
telescope based on the output of high resolution, on-axis
encoders, with the motion determined by a servo algorithm.
There are no gears, friction rollers, belts, or any other
mechanical reduction devices to introduce periodic errors or
compliance that could reduce the telescope’s stiffness and
natural frequency. As noted in Bely’s (2003) classic book,
The Design and Construction of Large Optical Telescopes, “A
direct drive, which eliminates all mechanical systems, is the
ultimate choice.”
While the stators, rotors, and rather
computer-controlled electronics are all commercially available,
they can be more expensive than many some modest-aperture
telescopes. Initiative member Dave Rowe devised a very low
cost, axial flux, direct drive motor for
telescopes. Normal direct drive motors are radial flux
motors. The radial “one ring inside another” configuration is
energy efficient, but involves an expensive arrangement of
magnets, coils, and soft steel to contain the flux. Rowe
realized that energy efficiency was not important for modest
aperture alt-az telescopes, and devised an axial flux
motor, with a simple coil ring placed on top of a
permanent magnet ring. The axial flux direct drive motor only
cost about $300 per motor in magnets, wire, and steel, and is
extremely easy to build.
 |
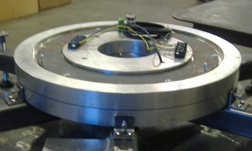 |
The axial-flux direct drive motor devised by Initiative
member Dave Rowe. An 18-inch diameter motor was readily
assembled from parts and materials that cost only $300.
The azimuth assembly on the right includes the
motor, bearings, and high resolution tape encoder and
read head. |
Reference: 18 Direct Drive Telescope - Cal Poly (Word doc)
click here
AC Synchronous Servo Motor Controller
Direct-drive, direct-position-reading servo
systems are much more difficult to control than ordinary brushed
DC motors, and require not only high resolution on-axis encoders
as inputs, but high-speed, computation of the control system
filters a feedback algorithm. Initiative member Dan Gray at
Sidereal Technology designed a low cost control system that
handles two “brushless,” AC synchronous, direct drive telescope
motors. Gray also wrote all of the real-time, embedded firmware
for the controller, and the PC-based command-and-control
software.
 |
The low cost direct drive servo motor controller devised
by Initiative member Dan Gray. An on-board
microcomputer performs the requisite trigonometric and
other calculations at high speed to control the current
through the motor’s coils. |
Reference: 18 Direct Drive Telescope - Cal Poly (Word doc)
click here
Back to Top
Optical Designs
Spherical Corrector
One of the early conclusions of the Alt-Az Initiative was that
one approach to keeping mirror costs and weight down beyond 1
meter aperture was to utilize multiple spherical primary
mirrors. For instance, four 0.75 meter primary mirrors
have an equivalent aperture of 1.5 meters, while four 1.0 meter
mirrors are equivalent to 2.0 meters, quite a respectable research
telescope. Telescopes with spherical primaries and instruments
at prime focus are of special interest to Initiative members not
only because spherical primaries are relatively easy to make
but, more importantly, because a number of smaller spherical
primaries can be grouped together to form a much larger
spherical primary—one that could potentially weigh and cost less
even when one considers the complications of multiple mirror
alignments.
The catch, of course, is that the
individual mirrors need to be tip-tilted with some precision,
and very significant spherical aberration needs to be
corrected. While aspheric secondary mirrors, such as
Pressman-Carmichael, could be employed, a lower cost (albeit
narrower field of view) approach is a refractive corrector with
the instruments at prime focus. Dave Rowe designed a two
element, all spherical surface, prime focus refractive corrector
for an effective 1.5 meter, f/3.5 telescope with a single
spherical primary mirror or multiple primary mirrors.
Corrected Dall Kirkham
Dave Rowe designed the optics for a 0.7 meter (28 inch)
corrected Dall Kirkham optics for a telescope with a Nasmyth
focus. This design employs a spherical convex secondary mirror
that is much less sensitive to alignment errors than
non-spherical secondaries. The two-element refractive all
spherical surfaces corrector provides a wide, crisp field with
generous back focus. This optical design is being incorporated
in the telescope that will be produced by PlaneWave Instruments.
This optical design and the
Corrected Hyperbolic Newtonian (CHN) design below were developed by
an automated ray-tracing program, Darwin, which Dave Rowe
developed. As with biological evolution, random variations are
introduced and the best results from each generation are allowed
to propagate, while less favorable variations fall by the
wayside. Dave has been known to run a design problem
through Darwin for
a week or two on a high speed PC.
Reference: Portable CDK Alt-Az Telescope (pdf)
click here
Corrected Hyperbolic Newtonian
Initiative members Dave Rowe and Tong Liu designed an optical
system for Corrected Hyperbolic Newtonian (CHN) telescopes. Their CHN design combines a generous back focus (not readily
available with parabolic primary correctors) with a wide, crisp
field of view. Their initiative concentrated on the design and
production of a two element, all spherical surfaces, refractive
corrector. A convenient focal length was chosen that resulted
in alt-az telescopes with a convenient eyepiece/instrument
height. Corrector lens sets are available from Hubble Optics as
are matching lightweight hyperbolic primary mirrors.
Reference: Aerospace Alt-Az Telescopes (pdf)
click here
Back to Top
Lightweight
Primary Mirrors
Both large
mountaintop alt-az telescopes and their diminutive lowland
brethren share a common obsession with lightweight primary
mirrors. Without lightweight mirrors, telescope weights and
hence costs rapidly get out of hand. For small telescopes,
especially in the 1-2 meter aperture range, transportability and
assembly are vital issues if 18-wheelers and cranes are to be
avoided. Initiative members have been addressing the challenges
of lightweight yet low cost primary mirrors for small alt-az
research telescopes from several perspectives.
Foam Glass Mirrors
Several Initiative members have been investigating the use of
foam glass as lightweight spacer/structural material between the
top and bottom glass plates of a primary mirror. Pittsburgh
Corning makes Foamglas, a rigid insulating material used in
LNG
ships, many industrial applications, and even under heated
concrete runways in cold climates such as Alaska. Andrew
Auregema has been machining concave surfaces in the tops of
Foamglas blocks, while David Davis has been slumping and fusing
glass plates to Foamglas in his kilns. Attendees at the recent
Galileo’s Legacy conference in Hawaii were intrigued when David
pointed out that Foamglas sandwich mirrors float on water. He
then proceeded to toss a Foamglas mirror blank on the floor
without damage. Soda lime Foamglas comes in a number of
densities, and there is also a low coefficient of temperature
expansion borosilicate version of Foamglas.
 |
Initiative member Andrew Aurigema machined the top
surface of Foamglas® material from
Pittsburgh
Corning. David Davis then fused thin glass plates to the Foamglas to
form a lightweight mirror blank. |
Initiative
members are investigating the use of low shrinkage adhesives to
fasten pre-slumped top plates (and flat bottom plates) to
Foamglas cores as an alternative to kiln fusion. A group in
Italy under the direction of Giovanni Pareschi is also working
on Foamglas mirrors, and the Director of Development at
Pittsburgh Corning, Steve Badger, has been generously supportive
of these Foamglas mirror developments.
Carbon Fiber Composite Replica Mirrors
Initiative member Peter Chen has been developing very
lightweight carbon fiber replica mirrors for several
years and
is achieving success with this approach.
Reference: Aerospace Alt-Az Telescopes (pdf)
click here |
 |
Polyurethane Replica Mirrors
Initiative member Kiran Shah is experimenting with polyurethane
replica mirrors. These mirrors have a solid polyurethane front
surface supported by a dense polyurethane foam body that is
formed in a mold. A concave master is pressed against
successive thin layers of solid polyurethane until its surface
is a reasonable inverse replica of the master. This work is
highly experimental, and Kiran does not expect high optical
quality. However, the cost to make these mirrors in volume
could be extraordinarily low, and thanks to their very light
weight, a number of spherical replica mirrors could potentially
be assembled into a multiple mirror telescope with an instrument
and refractive spherical corrector at prime focus. The
“instrument” might consist of a near infrared photodiode, a high
speed photodiode, or a fiber feed to a low resolution
spectrograph.
Meniscus, Sandwich, and Cast
Mirrors
David Davis has been experimenting with slumped meniscus
mirrors. He builds his own kilns and does his own
grinding, polishing, and figuring by machine. Both low
cost float glass and Pyrex have been slumped. Work is
also continuing on the development of sandwich mirrors
with glass spacers (Tong Liu at Hubble Optics), and with
cast mirrors with glass honeycomb backing structures
(Cary Chelborad and Allan Keller at Optical Structures).
Reference: 18 Direct Drive Telescope - Cal Poly (Word
doc)
click here |
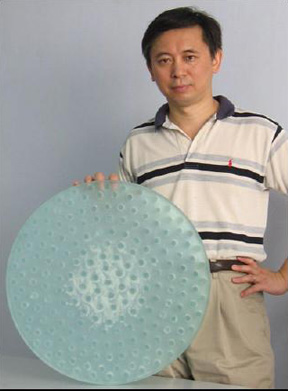 |
Back to Top
Active
Primary Mirror Control
Initiative members Mel Bartels and David Davis are both
interested in seeing how far thin, slumped meniscus mirrors can
be “pushed” with respect to both aperture and thinness. Soda
lime or borosilicate float glass can only be procured in
thicknesses up to 25 mm. As apertures of meniscus mirrors this
thin increase, however, an aperture will eventually be reached
where, similar to the situation with mountaintop alt-az
telescopes, corrective forces under computer control will need
to be applied to the primary mirror if it is to retain its
proper figure as the telescope changes its altitude and hence
the gravity vector acting on the mirror.
Voice Coil Active Primaries
In a visit to the Gemini North eight meter
telescope, Initiative members discussed the Gemini’s “voice
coil” active mirror support system with Chris Carter, then
Gemini’s control system engineer (Chris recently joined the
Thirty Meter Telescope developmental team). Chris suggested
that for smaller telescopes, a voice coil support system to
“tweak” the mirror into proper shape as altitude changed could
be made at low cost. Two undergraduate electrical engineering
students at
California Polytechnic State University are now developing low
cost electronic controls for voice coil meniscus mirror
adjustment. They are also, in a parallel effort, developing the
electronics for small stepper motor mirror tweaking.
.
|
 |
Chris Carter and Russell Genet examine one of the
Gemini’s spare voice coil actuators used to maintain the
shape of Gemini’s actively corrected primary mirror.
Chris suggested that active mirror support would be a
good tech transfer candidate. |
Reference: Big Island Discussion (Word doc)
click here
Stepper Active Primaries
The Cal Poly electrical engineering students are also, in a parallel effort, developing the
electronics for small stepper motor mirror tweaking. In both
cases, the concern is only for very low frequency (less than
once per second) adjustment of the primary mirror to correct for
the lowest order Zernike terms, especially astigmatism. The
requisite adjustments can, we believe, be made “off line” on a
number of bright stars distributed in altitude with a
coefficient determination algorithm that minimizes the spread of
the stellar image. During operation, the current altitude of
the telescope is noted, and the required settings interpolated
from a lookup table. This approach is used by the eight meter
Subaru telescope with recalibration of their table only required
about once a year.
References:
Bely, Pierre Y. 2003. The Design and
Construction of Large optical Telescopes.
New York: Springer.
Wilson, R.N. 1999. Reflecting Telescope
Optics II: Manufacturing, Testing, Alignment,
Modern Techniques. Corrected 2nd
printing 2001. Berlin: Springer.
Semi-passive Bimorph Mirror Correction
This initiative is just getting underway.
The objective is to provide corrections as a function of
telescope altitude to compensate for optical distortions
introduced from flexible mirrors or mirror support structures.
It is hoped that such corrections can be provided at low
frequency (less than once per second) via interpolation of
correction coefficients determined off line and occasionally
updated. Semi-passive bimorph mirrors are not expensive, and
such correction might provide a low cost alternative to heavier
mirrors or mirror supports.
Finally, there is a third approach we are considering for
active optical correction, and this is the use of a semi-passive
bimorph mirror. These mirrors consist of two thin disks, one of
active piezo material coated with a thin layer of conductive
metal on each side, and the other a thin front surface mirror.
The two disks are bonded together with a low shrinkage
adhesive. The back metal coating on the piezo disk can be
divided into sections. When a high voltage (100 to 350 volts)
are applied to the sections, the piezo materials in each section
contracts or expands some amount, depending on the applied
voltage and its polarity. The combination of these expansions
and contractions can warp the thin mirror disc in a modal
manner. A computer controls these voltages through a
digital-to-analog converters and high voltage operational
amplifiers.
Instruments
Bimorph Mirrors
Instead of using actuators to apply forces directly to the
primary mirror itself, a much smaller, semi-passive bimorph
mirror near the instrumental payload is distorted via applied
voltages in such a manner as to cancel out the gravity-induced
distortions in a lightweight primary mirror. These corrections
can be applied in a manner similar to what was discussed above,
i.e. via a lookup table versus altitude
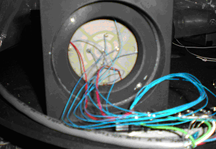
Initiative member Greg Jones’ low cost, semi-passive bimorph mirror. |
Instrument
Clusters
One of the Alt-Az Initiative’s members, Gary Cole, has been
focused on the concept of developing lightweight automated
science instrument clusters to maximize the number of available
observing modes on a single telescope. This in turn multiplies
both the research and educational value of the facility.
 |
Instrument cluster developed by Gary Cole. Permanently
mounted, yet remotely switchable instruments increase
system versatility and, compared to mounting and
demounting instruments, greatly reduce the costs of
operation and maintenance. |
The core of this project has been the development of a
programmable, lightweight, 4 way instrument selector that can
tie together, both optically and mechanically, a complete suite
of science instruments including the new 50mm CCD cameras. This
device is just now entering production from Optec, Inc.
 |
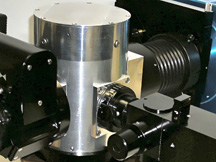 |
Left, 3-D drawing of the lightweight programmable
instrument selector that will be offered by Optec, Inc.
Right, bench testing of the
lightweight instrument cluster prototype. |
As an example, the prototype 20 pound cluster provides:
target acquisition, imaging, Sloan band photometry, deep R~400
survey spectroscopy, R~10000 spectroscopy, JH band infrared
photometry, and broadband dual beam imaging polarimetry. The
selector and instruments operate as IP network devices. The data
is gathered in FITS formats. A single laptop operates the
entire cluster along with the telescope under the control of an
automated observation scheduler.
Assuming a typical 1000 hr/year observing program and five
year lifetime, the amortized cost of this instrument cluster is
less than 5$/hour. The range of both research and education
opportunities provided by an instrument cluster such as this one
on a 1 meter platform is very exciting.
JH Band Photodiode Photometer
Automation
Currently, near infrared imaging cameras remain quite expensive
($50K and up) because these cameras cannot be fully implemented
in silicone chips alone, requiring a hybrid combination of
materials. While efforts are underway to reduce the cost of
these cameras, a much lower cost, and easier to handle option
for JH band near IR photometry is available in Optec’s SSP-4
photometer for only $3K. The SSP-4, developed by Optec, in
cooperation with the American Association of Variable Star
Observers, is ideally suited for observing long-period Mira and
other variable stars.
Initiative member Dan Gray has automated the filter changing
and flip-mirror functions of the Optec
SSP-4 to allow
fully automatic operation. Dan and Initiative member Russ Genet
made a test run on this modified
SSP-4 at the University of
Oregon’s
Pine Mountain Observatory in July 2008. Dave Rowe analyzed the
resultant data.
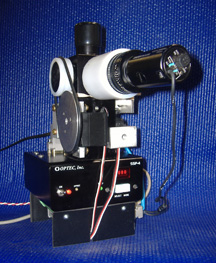 |
 |
The
Optec
SSP-4 near IR
photometer, left, modified for automated operation, was
evaluated on Dan Gray’s automated
14-inch alt-az
telescope (right) in a test run at
Pine
Mountain
Observatory. |
References:
Bely, Pierre Y. 2003. The Design and
Construction of Large optical Telescopes.
New York: Springer.
Wilson, R.N. 1999. Reflecting Telescope
Optics II: Manufacturing, Testing, Alignment,
Modern Techniques. Corrected 2nd
printing 2001. Berlin: Springer.
|